CO2 conversion into value-added chemicals and renewable fuels is one of the great challenges of the 21st Century, as it might contribute to a solution for the problem of global warming and it also reduces our dependence on fossil fuels. Plasma technology is very promising as energy-efficient alternative to the existing classical conversion methods, because the splitting of the inert molecules is initiated by energetic electrons present in the plasma. Moreover, plasma uses electricity and can easily be switched on/off, so it has great potential for temporary storage of excess renewable energy, which is also considered as one of the major challenges of this century. Thus, plasma has the potential to simultaneously solve 3 major problems: (i) global warming, (ii) our dependence on fossil fuels, and (iii) the transition to renewable energy. In addition, by combining the plasma with a catalyst, in so-called “plasma catalysis”, the selective production of value-added chemicals can be targeted. However, more fundamental research is crucially needed, to better understand the underlying mechanisms, in order to improve the conversion and energy efficiency of plasma technology. For this reason, we perform computer modelling as well as experiments. We model the plasma chemistry by 0D chemical kinetics modelling, focussing among others on the role of CO2 vibrational levels versus thermal conversion, as well as on mixtures of CO2 with CH4, H2O, H2 and N2. Furthermore, we also model various plasma reactors (i.e., dielectric barrier discharges (DBDs) and packed bed DBDs, microwave plasmas, gliding arc and atmospheric pressure glow discharges) by fully coupled 3D-axisymmetric and 2D-fluid dynamics models, to improve the design for energy-efficient CO2 conversion and investigate the effect of post-plasma gas quenching. Finally, we also model plasma-catalyst interaction (i.e., penetration of plasma species inside catalyst pores, and the chemical reactions at the catalyst surface. Our experiments are performed in various types of plasma reactors: cylindrical gliding arc plasmas (gliding arc plasmatron with reverse vortex flow, rotating gliding arc, …), microwave plasmas, atmospheric pressure glow discharges, pin-to-pin arc plasmas, and (packed bed) DBDs.
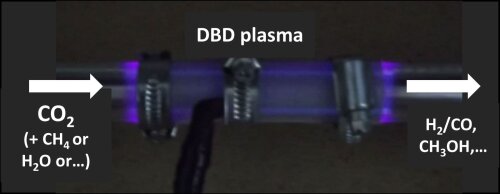
Moreover, besides CO2 conversion, plasma is also gaining increasing interest for CH4 conversion into either H2 (and value-added carbon) or into olefins, like ethylene (C2H4) and acetylene (C2H2), which are of great interest for the chemical industry. In addition, plasma can also be important for H2 synthesis from other hydrocarbons (e.g., methanol, ethanol, and even plastic waste pyrolysis products), as well as from NH3. We also perform research for these applications, again by a combination of plasma chemistry and plasma reactor modeling, and experiments.
Finally, plasma is also very promising for nitrogen fixation, i.e., the conversion of nitrogen from the atmosphere into chemical building blocks for all living organisms. This is a very important process in sustainable chemistry. Indeed, nitrogen is needed for the biosynthesis of all basic building blocks of plants and living organisms, such as amino acids, peptides and nucleic acids. There is more than enough nitrogen present in our atmosphere for this purpose, but these N2 molecules are very stable, and a lot of energy is required to break the triple bond between the N-atoms. Hence, it is important to develop energy-efficient processes for nitrogen fixation, where nitrogen from the atmosphere is converted into simple nitrogen compounds, like ammonia (NH3) or nitrogen oxides (NO, NO2), which can more easily be used as precursors for the biosynthesis of more complex molecules. Plasma is gaining increasing interest for the activation of nitrogen molecules in an energy-efficient way. Moreover, as mentioned above, plasma can be used for the storage of excess renewable energy, and it is very modular. Hence, this process also has great potential to be used at remote locations, for the local production of fertilizers by means of plasma, simply using air, in combination with solar or wind energy. However, more research is needed to further improve the energy efficiency of this process.
Again, we are developing models and performing experiments in various types of plasma reactors, for better insight and improving the performance.
Overall, plasma is very interesting for sustainable chemistry, i.e., the electrification of various chemical reactions, and we are exploring various applications.
Key publications
Plasma-assisted NH3 cracking in warm plasma reactors for green H2 production.
I. Fedirchyk, I. Tsonev, R. Quiroz Marnef and A. Bogaerts
Chem. Eng. J., 499, 155946 (2024) and supplementary material
Can post-plasma CH4 injection improve plasma-based dry reforming of methane? A modeling study.
M. Albrechts, I. Tsonev and A. Bogaerts
Green Chem., 26, 9712-9728 (2024) (2024 Green Chemistry Hot Article)
CO2 conversion to CO via plasma and electrolysis: A techno-economic and energy cost analysis.
J. Osorio-Tejada, M. Escriba-Gelonch, R. Vertongen, A. Bogaerts and V. Hessel
Energy Environ. Sci., 17, 5883 (2024)
Upscaling plasma-based CO2 conversion: Case study of a multi-reactor gliding arc plasmatron.
C. O’Modhrain, G. Trenchev, Y. Gorbanev and A. Bogaerts
J. Amer Chem Soc. Au, 4, 333-344 (2024) and supporting information
Coupled multi-dimensional modelling of warm plasmas: Application and validation for an atmospheric pressure glow discharge in CO2/CH4/O2.
S. Maerivoet, I. Tsonev, J. Slaets, F. Reniers and A. Bogaerts
Chem. Eng. J., 492, 152006 (2024)
Plasma catalysis in ammonia production and decomposition: Use it, or lose it?
Y. Gorbanev, I. Fedirchyk and A. Bogaerts
Curr. Opinion Green Sustainable Chem., 47, 100916 (2024)
Importance of plasma discharge characteristics in plasma catalysis: Dry reforming of methane vs. ammonia synthesis.
R. De Meyer, Y. Gorbanev, R.-G. Ciocarlan, P. Cool, S. Bals and A. Bogaerts
Chem. Eng. J., 488, 150838 (2024) and supporting information
Plasma-based dry reforming of CH4: Plasma effects vs. thermal conversion.
J. Slaets, B. Loenders and A. Bogaerts
Fuel, 360, 130650 (2024) and supporting information
Correction: From the Birkeland–Eyde process towards energy-efficient plasma-based NOx synthesis: A techno-economic analysis.
K.H.R. Rouwenhorst, F. Jardali, A. Bogaerts and L. Lefferts
Energy Environ. Sci., 16, 6170 (2023)
Avoiding solid carbon deposition in plasma-based dry reforming of methane.
O. Biodo, C.F.A.M. van Deursen, A. Hughes, A. van de Steeg, W. Bongers, M.C.M. van de Sanden, G. van Rooij and A. Bogaerts
Green Chemistry, 25, 10485 (2023)
Plasma-based CO2 conversion: How to correctly analyze the performance?
B. Wanten, R. Vertongen, R. De Meyer and A. Bogaerts
J. Energy Chem., 86, 180-196 (2023) and supplementary information I and supplementary information II
Is a catalyst always beneficial in plasma catalysis? Insights from the many physical and chemical interactions.
B. Loenders, R. Michiels and A. Bogaerts
J. Energy Chem., 85, 501-533 (2023) and supplementary information
Methane coupling in nanosecond pulsed plasmas: Correlation between temperature and pressure and effects on product selectivity.
E. Morais, E. Delikonstantis, M. Scapinello, G. Smith, G.D. Stefanidis and A. Bogaerts
Chem. Eng. J., 462, 142227 (2023) and supporting information
Nitrogen fixation by an arc plasma at elevated pressure to increase the energy efficiency and production rate of NOx.
I. Tsonev, C. O’Modhrain, A. Bogaerts and Y. Gorbanev
ACS Sustainable Chem. Eng., 11, 1888−1897 (2023) and supporting information
The 2022 Plasma Roadmap: Low temperature plasma science and technology.
I. Adamovich, S. Agarwal, E. Ahedo, L.L. Alves, S. Baalrud, N. Babaeva, A. Bogaerts, A. Bourdon, P.J. Bruggeman, C. Canal, E.H. Choi, S. Coulombe, Z. Donkó, D.B. Graves, S. Hamaguchi, D. Hegemann, M. Hori, H.-H. Kim, G.M.W. Kroesen, M.J. Kushner, A. Laricchiuta, X. Li, T.E. Magin, S. Mededovic Thagard, V. Miller, A.B. Murphy, G.S. Oehrlein, N. Puac, R.M. Sankaran, S. Samukawa, M. Shiratani, M. Šimek, N. Tarasenko, K. Terashima, E. Thomas Jr., J. Trieschmann, S. Tsikata, M.M. Turner, I.J. van der Walt, M.C.M. van de Sanden and T. von Woedtke
J. Phys. D: Appl. Phys., 55, 373001 (2022)
Foundations of plasma catalysis for environmental applications.
A. Bogaerts, E.C. Neyts, O. Guaitella and A.B. Murphy
Plasma Sources Sci. Technol., 31, 053002 (2022)
Effusion nozzle for energy-efficient NOx production in a rotating gliding arc plasma reactor.
S. Van Alphen, H. Ahmadi Eshtehardi, C. O’Modhrain, J. Bogaerts, H. Van Poyer, J. Creel, M.-P. Delplancke, R. Snyders and A. Bogaerts
Chem. Eng. J., 443, 136529 (2022) and its supporting information
Energy-efficient small-scale ammonia synthesis process with plasma-enabled nitrogen oxidation and catalytic reduction of adsorbed NOx.
L. Hollevoet, E. Vervloessem, Y. Gorbanev, A. Nikiforov, N. De Geyter, A. Bogaerts and J.A. Martens
ChemSusChem, 2022, e202102526 (2022) and its supporting information
Carbon bed post-plasma to enhance the CO2 conversion and remove O2 from the product stream.
F. Girard-Sahun, O. Biondo, G. Trenchev, G. van Rooij and A. Bogaerts
Chem. Eng. Jour., 442, 136268 (2022) and its supporting information
Dry reforming of methane in an atmospheric pressure glow discharge: Confining the plasma to expand the performance.
B. Wanten, S. Maerivoet, C. Vantomme, J. Slaets, G. Trenchev and A. Bogaerts
J. CO2 Util.,56, 101869 (2022) and its supporting information.
Oxygenate production from plasma-activated reaction of CO2 and ethane.
A.N. Biswas, L R. Winter, B. Loenders, Z. Xie, A. Bogaerts and J.G. Chen
ACS Energy Lett., 7, 236-241 (2022) and its supporting information.
Nitrogen fixation in an electrode-free microwave plasma.
S. Kelly and A. Bogaerts
Joule, 5, 3006-3030 (2021) and its supporting information.
Plasma catalysis for ammonia synthesis: A microkinetic modeling study on the contributions of Eley−Rideal reactions.
Y. Engelmann, K. van ’t Veer, Y. Gorbanev, E.C. Neyts, W. F. Schneider and A. Bogaerts
ACS Sust. Chem. Eng., 9, 13151−13163 (2021) and its supporting information.
Selective oxidation of CH4 to CH3OH through plasma catalysis: Insights from catalyst characterization and chemical kinetics modelling.
Y. Yi, S. Li, Z. Cui, Y. Hao, Y. Zhang, L. Wang, P. Liu, X. Tu, X. Xu, H. Guo and A. Bogaerts
Appl. Cat. B: Env., 296, 120384 (2021)
From the Birkeland–Eyde process towards energy-efficient plasma-based NOX synthesis: a techno-economic analysis.
K.H.R. Rouwenhorst, F. Jardali, A. Bogaerts and L. Lefferts
Energy Environ. Sci., 14, 2520 (2021) Correction published in 2023: Energy Environ. Sci., 16, 6170 (2023)
Sustainable gas conversion by gliding arc plasmas: A new modelling approach for reactor design improvement.
S. Van Alphen, F. Jardali, J. Creel, G. Trenchev, R. Snyders and A. Bogaerts
Sust. Energy Fuels, 5, 1786 (2021)
NOx production in a rotating gliding arc plasma: Potential avenue for sustainable nitrogen fixation.
F. Jardali, S. Van Alphen, J. Creel, H.A. Eshtehardi, M. Axelsson, R. Ingels, R. Snyders and A. Bogaerts
Green Chem., 23,1748 (2021) and its supporting information
Spatially and temporally non-uniform plasmas: Microdischarges from the perspective of molecules in a packed bed plasma reactor.
K. van ‘t Veer, S. van Alphen, A. Remy, Y. Gorbanev, N. De Geyter, R. Snyders, F. Reniers and A Bogaerts
J. Phys. D: Appl. Phys., 54, 174002 (2021)
On the kinetics and equilibria of plasma-based dry reforming of methane.
Y. Uytdenhouwen, K.M. Bal, E.C. Neyts, V. Meynen, P. Cool and A. Bogaerts
Chem. Eng. J., 405 126630 (2021)
How gas flow design can influence the performance of a DBD plasma reactor for dry reforming of methane.
Y. Uytdenhouwen, J. Hereijgers, T. Breugelmans, P. Cool and A. Bogaerts
Chem. Eng. J., 405, 126618 (2021)
Towards green ammonia synthesis through plasma-driven nitrogen oxidation and catalytic reduction.
L. Hollevoet, F. Jardali, Y. Gorbanev, J. Creel, A. Bogaerts and J.A. Martens
Angew. Chem. Int. Ed., 59, 23825-23829 (2020)(Hot paper, with publicity in ChemistryViews)
Plasma-driven catalysis: green ammonia synthesis with intermittent electricity.
K.H.R. Rouwenhorst, Y. Engelmann, K. van ‘t Veer, R.S. Postma, A. Bogaerts and L. Lefferts
Green Chem., 22, 6258 (2020)
Plasma-based CO2 conversion: To quench or not to quench?
V. Vermeiren and A. Bogaerts
J. Phys. Chem. C, 124, 18401-18415 (2020)
The 2020 plasma catalysis roadmap.
A. Bogaerts, X. Tu, J.C. Whitehead, G. Centi, L. Lefferts, O. Guaitella, F. Azzolina-Jury, H. Kim, A.B. Murphy, W.F. Schneider, T. Nozaki, J.C. Hicks, A. Rousseau, F. Thevenet, A. Khacef and M. Carreon
J. Phys. D: Appl. Phys., 53, 443001 (2020)
Plasma-based N2 fixation into NOx: Insights from modeling toward optimum yields and energy costs in a gliding arc plasmatron.
E. Vervloessem, M. Aghaei, F. Jardali, N. Hafezkhiabani and A. Bogaerts
ACS Sustainable Chem. Eng., 8, 9711-9720 (2020) and its supporting information
Plasma technology for CO2 conversion: A personal perspective on prospects and gaps.
A. Bogaerts and G. Centi
Front. Energy Res., 8, 111 (2020)
Predicted influence of plasma activation on nonoxidative coupling of methane on transition metal catalysts.
Y. Engelmann, P. Mehta, E.C. Neyts, W.F. Schneider and A. Bogaerts
ACS Sustainable Chem. Eng., 8, 6043−6054 (2020) and its supporting information
Dual-vortex plasmatron: a novel plasma source for CO2 conversion.
G. Trenchev and A. Bogaerts
J. CO2 Utilization, 39, 101152 (2020)
CO2 and CH4 conversion in “real” gas mixtures in a gliding arc plasmatron: how do N2 and O2 affect the performance?
J. Slaets, M. Aghaei, S. Ceulemans, S. Van Alphen and A. Bogaerts
Green Chem., 22, 1366 (2020)
Nitrogen fixation with water vapor by nonequilibrium plasma: Towards sustainable ammonia production.
Y. Gorbanev, E. Vervloessem, A. Nikiforov and A. Bogaerts
ACS Sustainable Chem. Eng., 8, 2996-3004 (2020)
Plasma technology - a novel solution for CO2 conversion?
R. Snoeckx and A. Bogaerts
Chem. Soc. Rev., 46, 5805-5863 (2017) (Paper featered on the back cover page of the journal)
Plasma technology: An emerging technology for energy storage.
A. Bogaerts and E.C. Neyts
ACS Energy Lett., 3, 1013-1027 (2018) (Invited feature article and selected to be featured in ACS Editors' Choice + Free Open Access)
Atmospheric pressure glow discharge for CO2 conversion: Model-based exploration of the optimum reactor configuration.
G. Trenchev, A. Nikiforov, W. Wang, St. Kolev and A. Bogaerts
Chem. Engng. J., 362, 830-841 (2019)
Modeling plasma-based CO2 and CH4 conversion in mixtuires with N2, O2 and H2O: The bigger plasma chemistry picture.
W. Wang, R. Snoecks, X. Zhang, M.S. Cha and A. Bogaerts
J. Phys. Chem. C, 122, 8704-8723 (2018) and its supporting information. (Invited feature article and selected for the cover of the journal).
Novel power-to-syngas concept for plasma catalutic reforming coupled with water electrolysis.
K. Li, J.-L. Liu, X.-S. Li, H.-Y. Lian, X. Zhu, A. Bogaerts and A.-M. Zhu
Chem. Engng. J., 353, 297-304 (2018)
Plasma streamer propagation in structured catalysts.
Q-Z. Zhang and A. Bogaerts
Plasma Sources Sci. Technol., 27, 105013 (2018)
Propagation of a plasma streamer in catalyst pores.
Q.-Z. Zhang and A. Bogaerts
Plasma Sources Sci. Technol., 27, 035009 (2018)
Effect of plasma-induced surface charging on catalytic processes: application to CO2 activation.
K.M. Bal, S. Huygh, A. Bogaerts and E.C. Neyts
Plasma Sources Sci. Technol., 27, 024001 (2018)
(Selected by the editors of Plasma Sources Science and Technology as one of the “Highlights of 2018" in the "Papers" section)
Dry reforming of methane in a gliding arc plasmatron: towards a better understanding of the plasma chemistry.
E. Cleiren, S. Heijkers, M. Ramakers and A. Bogaerts
ChemSusChem, 10, 4025-4036 (2017) and its supposting information. (Cover feature of the journal)
Gliding arc plasmatron: providing an alternativemethod for carbon dioxide conversion.
M. Ramakers, G. Trenchev, S. Heijkers, W. Wang and A. Bogaerts
ChemSusChem, 10, 2642-2652 (2017) and its supporting information.
Plasma technology: An emerging technology for energy storage.
A. Bogaerts and E.C. Neyts
ACS Energy Lett., 3, 1013-1027 (2018) (Invited feature article and selected to be featured in ACS Editors' Choice + Free Open Access)
Nitrogen fixation by gliding arc plasma: better insight by chemical kinetics modelling.
W. Wang, B. Patil, S. Heijkers, V. Hessel and A. Bogaerts
ChemSusChem, 10, 2145-2157 (2017) and its supporting information. (Paper featered on the cover page of the journal, as "cover profile" and "very important paper" of ChemSusChem).
The quest for value-added products from carbon dioxide and water in a dielectric barrier discharge: a chemical kinetics study.
R. Snoeckx, A. Ozkan, F. Reniers and A. Bogaerts
ChemSusChem, 10, 409-424 (2017) and its supporting information.
CO2 conversion in a dielectric barrier discharge plasma: N2 in the mix as a helping hand or problematic impurity?
R. Snoeckx, S. Heijkers, K. Van Wesenbeeck, S. Lenaerts and A. Bogaerts
Energy Environm. Sci., 9, 999-1011 (2016)
Can plasma be formed in catalyst pores? A modeling investigation.
Y.-R. Zhang, K. Van Laer, E.C. Neyts and A. Bogaerts
Appl. Catal. B: Environm., 185, 56-67 (2016)
Plasma catalysis: synergistic effects at the nanoscale.
E.C. Neyts, K. Ostrikov, M.K. Sunkara and A. Bogaerts
Chem. Rev., 115, 13408-13446 (2015)
Carbon dioxide splitting in a dielectric barrier discharge plasma: a combined experimental and computational study.
R. Aerts, W. Somers and A. Bogaerts
ChemSusChem, 8, 702-716 (2015)
Plasma-based conversion of CO2: current status and future challenges.
A. Bogaerts, T. Kozák, K. Van Laer and R. Snoeckx
Faraday Discuss., 183, 217-232 (2015)
Splitting of CO2 by vibrational excitation in non-equilibrium plasmas: a reaction kinetics model.
T. Kozák and A. Bogaerts
Plasma Sources Sci. Technol., 23, 045004 (2014)(Selected by the editors of Plasma Sources Science and Technology as one of the “Highlights of 2014")